More Efficient
Posted : admin On 4/8/2022Being more efficient at work can help us achieve the ultimate work-life balance that we are all chasing. These tips can help you achieve your goals and learn new methods to be more organised within the workplace. What do you do to boost your efficiency at work? Join in on the conversation below to let us know what your tips and tricks are. That has me wondering: is it more energy- and cost-efficient to use a space heater for 8–10 hours while keeping the thermostat low, or does the wattage required by a space heater make up for the cost of running the heating system? The answer is: it depends. There are multiple factors to consider such as. Raising kids bilingual can make them more attentive and efficient as adults. Published Fri, Jan 22 2021 3:38 PM EST. Cory Stieg @corystieg.
- Unless you’re Jim Halpert and enjoy spending most of your time pranking Dwight, most of us want to become more efficient at work. Not only is this beneficial for your career, but it can also.
- Smaller, more efficient tokamak could follow in ITER’s fusion footsteps Physics modeling and design show SPARC feasibility, but uncertainties remain. Chris Lee - Oct 27, 2020 10:45 am UTC.
Renewable energy has been on the defensive recently. Following the release of Planet of the Humans, the controversial new climate change documentary executive produced by Michael Moore, fossil fuel–backed climate denial groups are bashing wind and solar power with renewed vigor, regurgitating the film’s flawed, ancient talking points about the supposed poor performance and unreliability of these energy sources.
Those talking points include the assertion that solar power is wildly inefficient, something director Jeff Gibbs demonstrates by visiting a solar farm in Michigan where photovoltaic panels convert “just under 8 percent” of the energy in sunlight to electricity. But that efficiency rating is, as the photovoltaic-focused publication PV Magazine puts it, “from another solar era”: Today’s typical silicon solar panels operate at around 22 percent efficiency. And a new crystalline material called perovskite could soon raise the solar efficiency bar much further.
Solar photovoltaic cells — the individual units that form a solar panel, like the shingles on a roof — are wafer-like devices made of materials called semiconductors that are capable of converting sunlight to electrical energy. But even the best semiconductors only capture a fraction of the light that strikes them. Sunlight spans a wide range of wavelengths, and depending on the properties of the semiconductor and the design of the cell, some proportion of that light is reflected, some passes through, and some is absorbed but converted into heat before the energy can be put to use. Designing new solar cells that convert a larger fraction of incoming sunlight into electrical energy, or that have a higher conversion efficiency in solar power parlance, is one of the most active areas of solar research today.
Get Grist in your inbox
Always free, always fresh
Ask your climate scientist if Grist is right for you. See our privacy policy
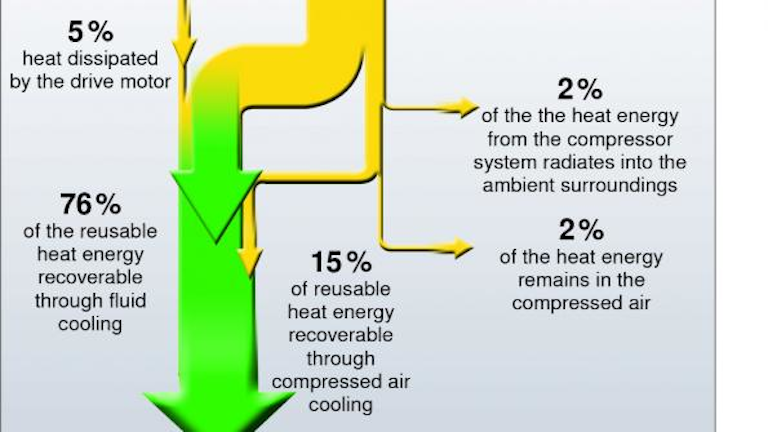
Perovskites first attracted the interest of the solar community a little over a decade ago, when scientists discovered this particular class of semiconductors has an outstanding ability to convert sunlight to energy. Today, perovskites are at the center of an effort to develop a new generation of thin-film solar cells that are cheaper than, and around four times as efficient as, the solar farm Gibbs visits in Planet of the Humans. The field is moving fast: In late April, the U.S. Department of Energy’s National Renewable Energy Laboratory announced it will be forming a U.S. Manufacturing of Advanced Perovskites Consortium (US-MAP) to accelerate the development of perovskite-based technologies.
According to US-MAP director Joseph Berry, the consortium will allow government researchers, academics, and private companies to pool resources and brainpower in order to tackle the biggest hurdle facing the commercialization of perovskite solar cells: Making these soft, easily degraded materials more durable.
“That’s where the challenge for this technology is,” Berry told Grist.
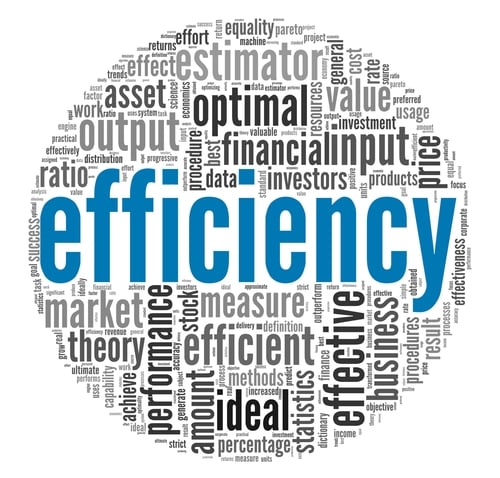
Perovskite refers to any compound that shares the same crystal structure as the mineral perovskite, also known as calcium titanate. The perovskites the solar industry is interested in aren’t mined from the Earth, but cooked up in labs. Inside these synthetic, or “hybrid,” perovskites, a mix of organic compounds, metals, and halides (reactive elements that include chloride, bromide, and iodide) sub in for calcium and titanium in the crystal lattice. Because of their unique structure and chemical composition, hybrid perovskites have a superpower: They’re remarkably good at absorbing sunlight. “They are kind of awesome in terms of their power conversion efficiency,” Berry said.
While silicon solar cells have a maximum potential efficiency of around 29 percent, a single perovskite layer can theoretically achieve efficiencies of closer to 33 percent. And by synthesizing perovskites that are sensitive to different parts of the solar radiation spectrum and stacking them to create a tandem cell, the efficiency can be boosted further — potentially above 40 percent, said Jao Van De Lagemaat, who directs the chemistry and nanoscience center at the National Renewable Energy Laboratory.
“That’ll take an enormous amount of engineering,” Van De Lagemaat said. But some researchers have already demonstrated that devices with two layers of perovskites are more efficient than a single perovskite solar cell, he said. Traditional silicon cells can be made more efficient by adding perovskites, too.
Not only are perovskites inherently better at harvesting sunlight than silicon, it’s potentially cheaper to mass-produce solar cells that rely on them. While silicon cells are manufactured via a complex process that involves purifying silicon from quartz in a high-temperature furnace, perovskites can be fabricated at low temperatures using far less energy, from cheap and readily available ingredients. Companies are already working on a variety of low-cost techniques for applying perovskites to a supporting surface, like a piece of glass, to turn that surface into a thin-film solar cell. These include inkjet printers, perovskite-based sprays, and roll-to-roll manufacturing techniques similar to those used for newspaper printing.
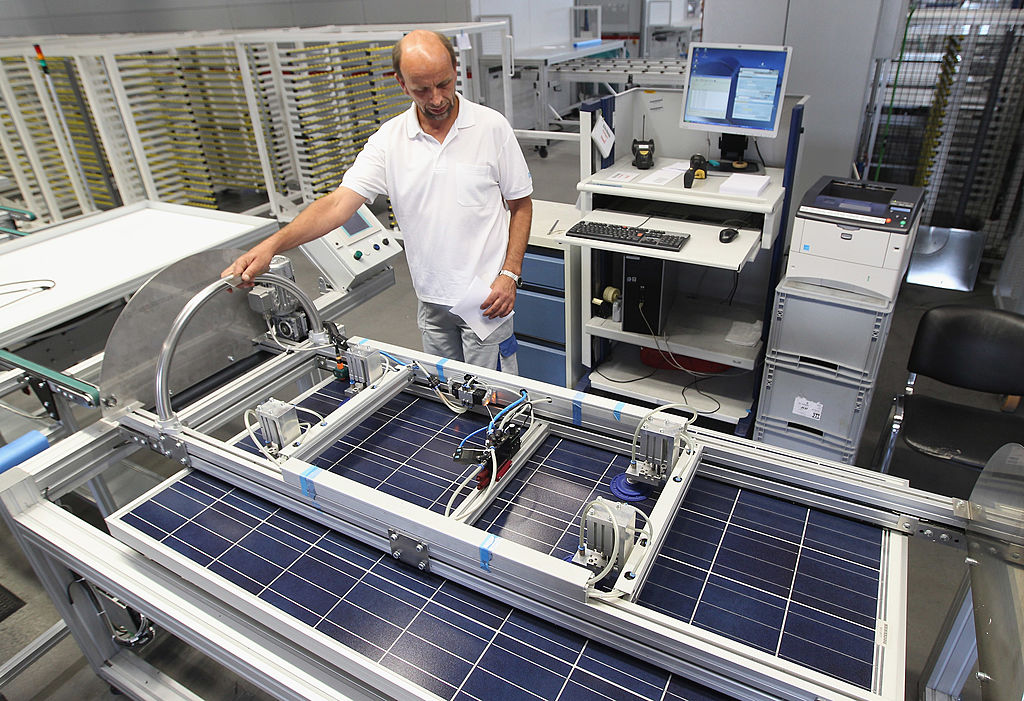
But despite all their appeal, you can’t buy a perovskite solar panel to put on your roof just yet. The reason? Easy-to-make perovskites are also easy to unmake.
“The material itself intrinsically is not stable,” said Letian Dou, an assistant professor of chemical engineering at Purdue University.
Perovskites dissolve in water, and they don’t hold up well under heat — both of which are a problem if you’re trying to manufacture a device to function atop a roof for decades. When a solar panel is heated by the sun, Dou says, its temperature can rise as high as 160 degrees F. At those temperatures, ions inside perovskites move around very quickly, causing the molecular structure to degrade. Even at room temperature, some “ion migration” can occur, creating instabilities in the material.
However, emerging research suggests perovskites can be stabilized by tweaking the chemical recipe. Recently, Dou and his colleagues doped perovskites with a rigid molecule called a ligand, allowing the material to remain stable at temperatures of up to 212 degrees F. The research, published last month in Nature, is preliminary — the tests were done at the lab scale, and on timescales of days, not decades — but it points to a “promising direction” for making commercially ready perovskites, Dou says. In a March study published in Science, scientists at the National Renewable Energy Laboratory made perovskites using three halide elements instead of the usual two, and found that the material degraded less than 4 percent after 1,000 hours of continuous operation in sunny conditions.
Berry says that when the Department of Energy (DOE) first started investigating perovskites in solar applications back in 2013, “lifetimes were measured on the order of hours, maybe tens of hours. And we’ve made a three orders of magnitude change in that. But the challenge is to make another couple of orders of magnitude change.”
The new US-MAP consortium aims to accelerate these efforts by enabling private companies, government researchers and academics to share new ideas and research facilities, and by encouraging them to join forces to conduct more ambitious tests. While all of the founding organizers are bringing their own research funds to the table, the consortium plans to seek additional funding from federal agencies like the DOE, as well as various state and local government programs and industry partners.
“The idea is we want to sort of band together,” Van De Lagemaat said. “There is quite a bit of research that can be done collectively that doesn’t really impact each individual company’s special sauce, but that really helps bring the entire area forward.”
Some of US-MAP’s commercial partners are already thinking about how to get the first perovskite solar panels out into the wild. Swift Solar, a California startup founded in 2017, is planning to stack two different layers of perovskites in tandem to create lightweight, high-efficiency solar cells that initially will be marketed for mobile applications like drones, satellites, and the lighting and air conditioning systems on trucks. Swift Solar co-founder Kevin Bush says vehicles are a good place for the first generation of perovskite solar panels to shine, both because portability is at a premium and because mobile markets “might only need five to 10 years’ stability, and it’s just a lot easier to promise that” right now.
“I think it’s nice to have markets that are initially not that demanding” in terms of longevity, Bush said, “so we can prove the technology out more.”
Van De Lagemaat doesn’t think perovskites will ever replace silicon wholesale in the solar power world. But he does think they will come to play “a very large role in the power market,” partly because rising solar efficiency and manufacturing improvements have caused the price of solar power to fall dramatically in recent years. While this price drop is good for consumers, it also means there’s less money to invest back into new silicon solar factories, which are expensive to build. Technologies that require less up-front capital investment, like perovskites, might ultimately be necessary to build the amount of solar power the world needs in the coming decades, he says.
If that hunch is correct, Planet of the Humans’ already outdated criticism of solar efficiency will soon feel prehistoric.
Sign up for The First 100 newsletter
Will Biden deliver on climate? Get weekly analysis of federal climate policy action in the first months of Biden’s term.
Depending on whom you ask, fusion power is either already here (but no one will purchase my sekrit design!), never going to happen (so stop wasting money!), or a difficult problem that might be a partial solution to an even more difficult problem. The last, being the view of scientists who actually work in the field, is often lost in all the noise.
Out of this fog of discussion, a passel of papers emerged recently, all focused on a proposed fusion project: the SPARC tokamak. One of the surprising things about SPARC is its size. Coming in at just over 3m across, SPARC will be smaller than currently operating tokamaks, like JET, which is nearly 6m across. ITER, currently under construction in France, is over 12m across. Yet SPARC and ITER are projected to have about the same performance. Both are expected to produce more energy from fusion than the direct input energy, though neither is expected to produce useful power.
So why the difference? And what does this latest batch of papers tell us about the design?
Molten magnets and mad safety officers
In tokamaks, how you use it doesn’t matter; size matters. These devices confine a hot plasma using a donut-shaped magnetic field. One of these two needs to be big for the tokamak to work. If you can make a stronger magnetic field, you can get away with a smaller tokamak and still get the same amount of output power.
More Efficient To Maintain Temperature
The magnetic fields are generated by coils that carry very large currents. Generating the current is not really a problem, but carrying it in a conductor is. If you use something like copper, then the power lost to the resistance of the wires will be huge, as power loss increases with the square of the current. Even worse, as the copper heats up, its resistance increases, leading to more heat generation, a shiny puddle of liquid copper, and a severely unimpressed safety officer.
Superconducting magnets are pretty much the only option. But all superconductors have a maximum current density. Exceed that and the conductor will start to show some resistance. Unfortunately, the materials from which superconductors are made are often poor normal conductors. Failing to obey the laws of physics here will lead to an apoplectically angry safety officer and a bespectacled accountant trying to bill you for a large amount of helium and, possibly, a new building.
Once you know the maximum current you can carry, the magnetic field can be determined. From that, you choose how much power you wish to generate via fusion, and that sets the size of the tokamak. (Approximately; there are a few missing details in this process.)
AdvertisementGiven this knowledge, and given that ITER was designed with state-of-the-art magnets, you’d have to conclude SPARC had trouble doing this math. Except ITER was designed in the 1990s and 2000s. Since then, high-temperature superconductors that have much better performance have been discovered and brought into commercial production. Using these modern superconductors, the magnetic-field strength can be doubled, allowing the size of the tokamak to be reduced considerably.
The future’s so bright?
While both ITER and SPARC are entering unknown territory, there is quite a bit of difference between the two projects. ITER has been modeled and studied to the nth degree. Teams of scientists have worked on every aspect for decades to try to predict the performance of ITER. SPARC, as a smaller device, cannot simply transfer the numbers and scale everything down by a factor of two. The recent papers try to address the challenge of modeling the new design.
They show that, fundamentally, SPARC seems sound. The plasma should reach the right conditions. The plasma should be able to maintain itself—it can carry a current that generates a magnetic field that helps confine itself—for about the same time as similarly sized tokamaks. That seems OK.
On the other hand, instabilities are likely to be exacerbated because the plasma is denser. In particular, phenomena called edge-localized modes could develop faster and be harder to suppress (or reduce). These instabilities occur at the edge of the plasma and, at worst, lead to hot plasma exhausting itself on the vessel walls. Other instabilities are disruptive in different ways, leading to reduced confinement and lower temperatures, so these generally need to be controlled.
These instabilities, if not controlled, can result in massive currents flowing in the vacuum vessel with extensive damage. This is the sort of scenario that gives ITER engineers nightmares, and the situation is not much different for SPARC: large currents, the whole machine jumping off its foundations, and other enjoyably dynamic disasters are possible. However, SPARC also seems to behave similarly to existing tokamaks, meaning that the predicted instabilities should be controllable.
A diverting puddle of tungsten
Where things really seem marginal is in the diverter. In every tokamak, there is a null point in the magnetic field. Particles don’t just leak through the null—they spray like a firehose. The diverter is the chosen place where this spray of particles hit a surface.
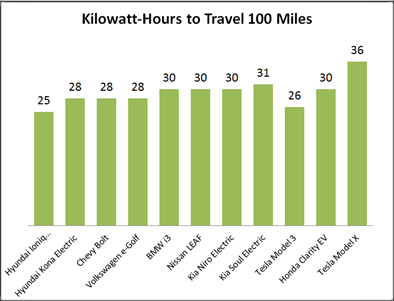
Even in current-generation tokamaks, the diverter materials don’t survive very long. In ITER, the diverter is going to be subject to conditions that are even more extreme. SPARC may make ITER look like warm milk.
Under their most pessimistic scenario, tungsten bricks will cyclically melt and recrystallize. During this process, tungsten atoms will probably penetrate to the core plasma, cooling it, and may even quench the fusion reaction. Carbon, an alternative, is a sacrificial surface that doesn’t kill the plasma. So carbon may end up being used in SPARC so that they can demonstrate that fusion works.
But the end result of using carbon will be organic molecules with a high percentage of tritium—not something to be messed around with. And definitely not something that should be considered for a commercial reactor.
AdvertisementEndgame
The success or failure of fusion rests on three pillars: science, engineering, and economics. ITER and SPARC will complete the science pillar. Assuming that we understand the physics pretty well—and I think we do—both tokamaks will show that fusion reactors that can produce useful electricity are possible. ITER will go a step further than SPARC and demonstrate many of the engineering requirements as well. Tokamaks will need to be cleaned robotically, for instance, and this is in the plan for ITER. The results from ITER can be transferred to future fusion reactors.
In any event, with sufficient work, the engineering case will probably be made. The economic case is much less certain. Most fusion researchers acknowledge this. The ITER-style large tokamak is a similar investment to the nuclear plants of the 1950s. And the payoff (if it ever arrives) is on a similar time scale. No one is sure who, if anyone, is prepared to risk that much money on that prospect. SPARC, by reducing the scale considerably, makes the economic case better by making the time scales shorter.
More Efficient Solar Panels
Weirdly, in retrospect, this makes the delays in ITER a lucky accident. Imagine if ITER had broken ground in 1990. By 2000, ITER would have been operating. The physics would have been worked out. The engineering path would have been clear. And the economics would have also been clear. In such an environment, the push for SPARC might never have come.
More Efficiently
Journal of Plasma Physics, 2020, Special Issue